So this post and a bunch after this one are from a talk I presented at the end December on the topic of lasers in space and astronomy. The target the audience was members from astronomy groups in the region with some knowledge of optics but not necessarily lasers and the curious people that might be interested but have a physics background from high school. I tried to make it interesting for a wide audience, and I would say that it is accessible to some degree to someone in high school, maybe earlier? Anyway, the goal was to be entertaining, it was, after all, happening during Christmas and new year vacation…
The first part was about the history of light and lasers, with a small chronological view of how we got to where we are today. Here we go!
The laser is a recent invention from the 60’s. But to get to the point of understanding what lasers are, we need to go further. And for the sake of the discussion, let’s go from the very beginning of civilization, where the concept of light and vision were still not fully understood.
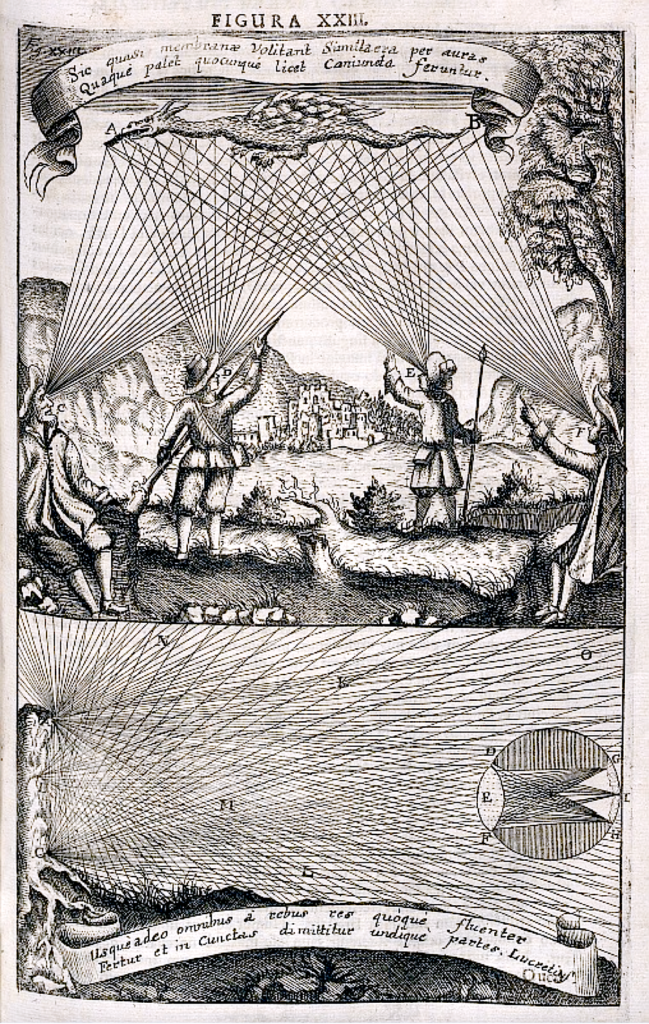
I will start then with the Greeks who were the first to record their thoughts on what vision was. For Pythagore and Plato [1], the act of seeing was simple. The eye, which is a creation of the gods, is composed of all the elements of the world: earth, fire, air and water. The fire in the eye emits particles of light that goes from the eye to the thing that people are looking at, in a straight line. This allows the viewer to get information on the shape, colour and distance of the object. Needless to say that this view is far from what actually happens.
Approximately 1000 years later comes Abu Ali Hasan Ibn Al-Haithan, who also went with the name of Alhazen. Alhazen is recognized by a lot of people as the first “optical physicist”, as he studied many optical phenomenons and tried to explain them, with more success than before [2]. For instance, he studied rainbows and mirages. He also got an interest in vision, and challenged the view from the Greek philosophers. Using simple logic actually, he demonstrated that light does not originate from the eye, but rather goes into the eye, in a straight line. Not bad. Actually, his work would be very important later, especially, his work would influence Kepler and other famous scientists.

In the 17th century, Huygens is going to think more about what is light exactly. For him, light is a wave, a little like a sound wave. That is to say that light is an oscillation of “something”. Now, that “something” was not fully understood, and at that time, it would be called “ether”, a concept that is going to stay alive until the 1900’s. All in all, this is a huge step in the understanding of light. But this is not all. At approximately the same time, Newton, another very famous scientist, was thinking about the same question, but came to a different conclusion. To Newton, light is actually a particle, like a small dust particle that travels from a point to another in a straight line. This discussion about the nature of light is a famous one, and is called the wave particle duality of light. Because Newton’s reputation at the time was “better” than Huygens, his idea won and from there, people accepted the fact that light was a particle.
In 1801, the theory from Huygens came back because of the experiments of another scientist: Thomas Young. Young created a simple experiment that proved that light was actually behaving as a wave. The experiment is famous now and is called the double-slit experiment. The idea is that a light source such as a candle or simply the sun is directed toward a wall on which 2 parallel thin slits are made. Behind the wall with the slits, we have another wall, which is the observation wall.
If light is composed of particles, the particles would go either in on slit or the other, and on the observation wall, we would only see a blob of light, with no particular shape. If, on the contrary, light is a wave, then the wave from the light source would go through both slits. Behind the wall, the waves from both slits would interfere and create an interference pattern on the observation wall. We would see dark lines alternating with bright lines. As it turns out, the result of the experiment is the presence of these interference lines, which is the sign that light is a wave! Case closed…
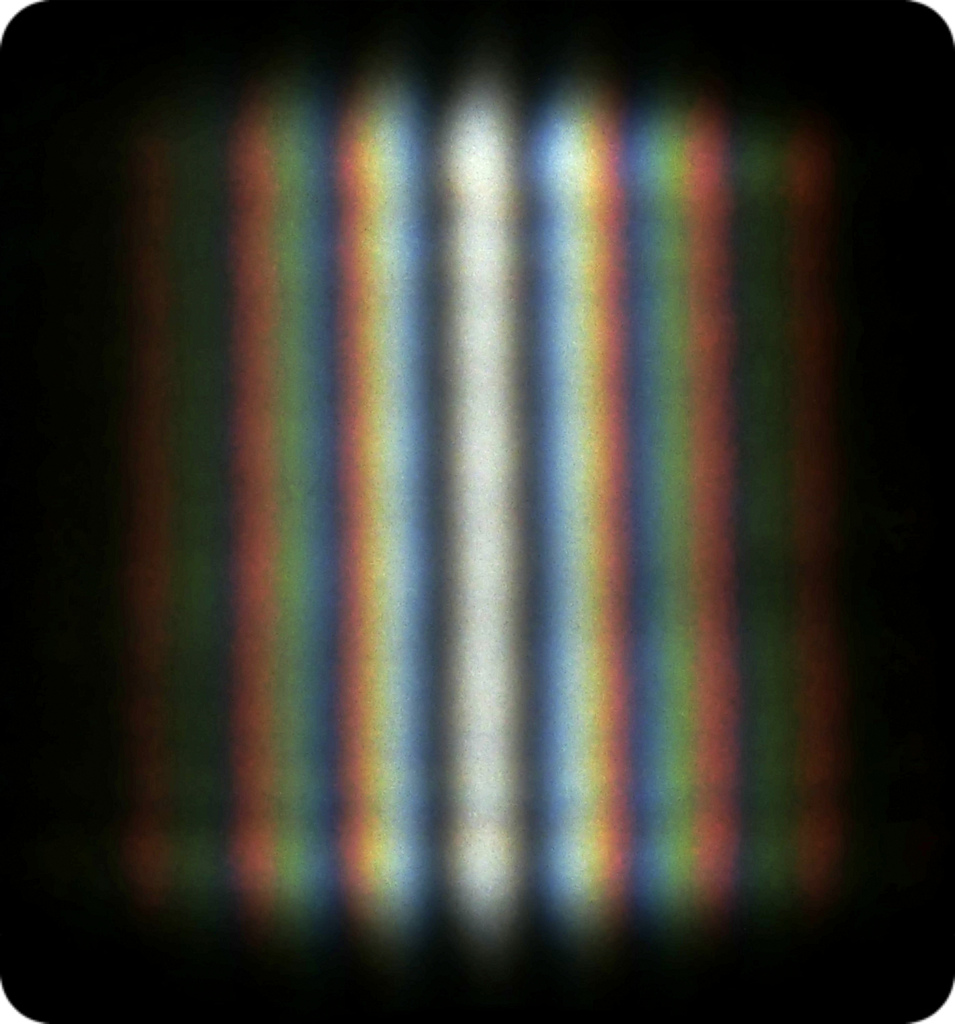
Just 50 years later, another famous name in physics and optics comes up: James C. Maxwell. Maxwell’s work revolved around electromagnetic waves and their mathematical descriptions. To this day, Maxwell’s equation are the way we describe “classical” light in mathematical terms. The main takeaway here is that thanks to this work, we know now that light is an electromagnetic wave, that is to say the oscillation of the electric and magnetic field together.
At the beginning of the 20th century, Plank and Einstein, other famous names of physics, double down on the strange nature of light. They take the particle approach from Newtown and the wave approach of Huygens and somehow manage to mix both interpretation together, making light both a wave and a particle. This particle/wave will be named “photon”. How can it work? Let’s take again the double slit experiment. A photon will be emitted by the light source. It is a single photon. This photon starts as a wave, propagating from the light source to the double slits and it will pass both slits at the same time. Behind the slits, the wave of the photon interferes and eventually, the interferences reach the observation wall. At that place, because we take a measurement, we can detect the photon in a single spot. The position of this spot depends on the interference pattern from the double slit, and some probability component. Because, if we take the same experiment and do it again, the photon will arrive on the observation wall at a different spot than the time before. Do it again, and it is yet another spot. Actually, if we do this experiment a million times, we eventually see that the spots on the observation wall will form the interference pattern, the bright and dark lines that Huygens was able to observe. See the great visualization there:
The photon is a quantum object that obeys the strange laws of the quantum world. Without going to much into the details, the point is that before “observation”, a quantum object is just a wave. What “observation” means is complicated. It can be measurement, it can be interaction with something else in a way or another. People are still figuring this out. If you were to monitor the slits, so as to know where the photon passes, you would be able to see the photon passing EITHER in the first OR in the second slit…. Isn’t that strange? Worst, the interference pattern disappears and we are left with a big blob of light in the observation wall. The only thing we did was to measure the photons at the slit wall, nothing else.
Let’s leave the quantum weirdness a few moments, and focus on an important characteristic of the photon: It’s energy. A photon will be created with a given finite amount of energy, which is going to exist from the start of the photon’s life to it’s destruction. During it’s life, the photon doesn’t lose or gain energy. Another interesting thing is that the energy from a photon is directly linked to it’s wavelength. It is easy to understand. Electromagnetic waves that oscillate slowly will have a big wavelength. It is for instance radio waves that can have a wavelength of several cm. Electromagnetic waves that are oscillating fast have more energy. Fast oscillations mean short wavelength, like a few hundred of nm, typically this is what are UV photons and why they are so dangerous for the skin. They have a lot of energy that can destroy DNA in the skin and cause unwanted mutations, aka cancer.
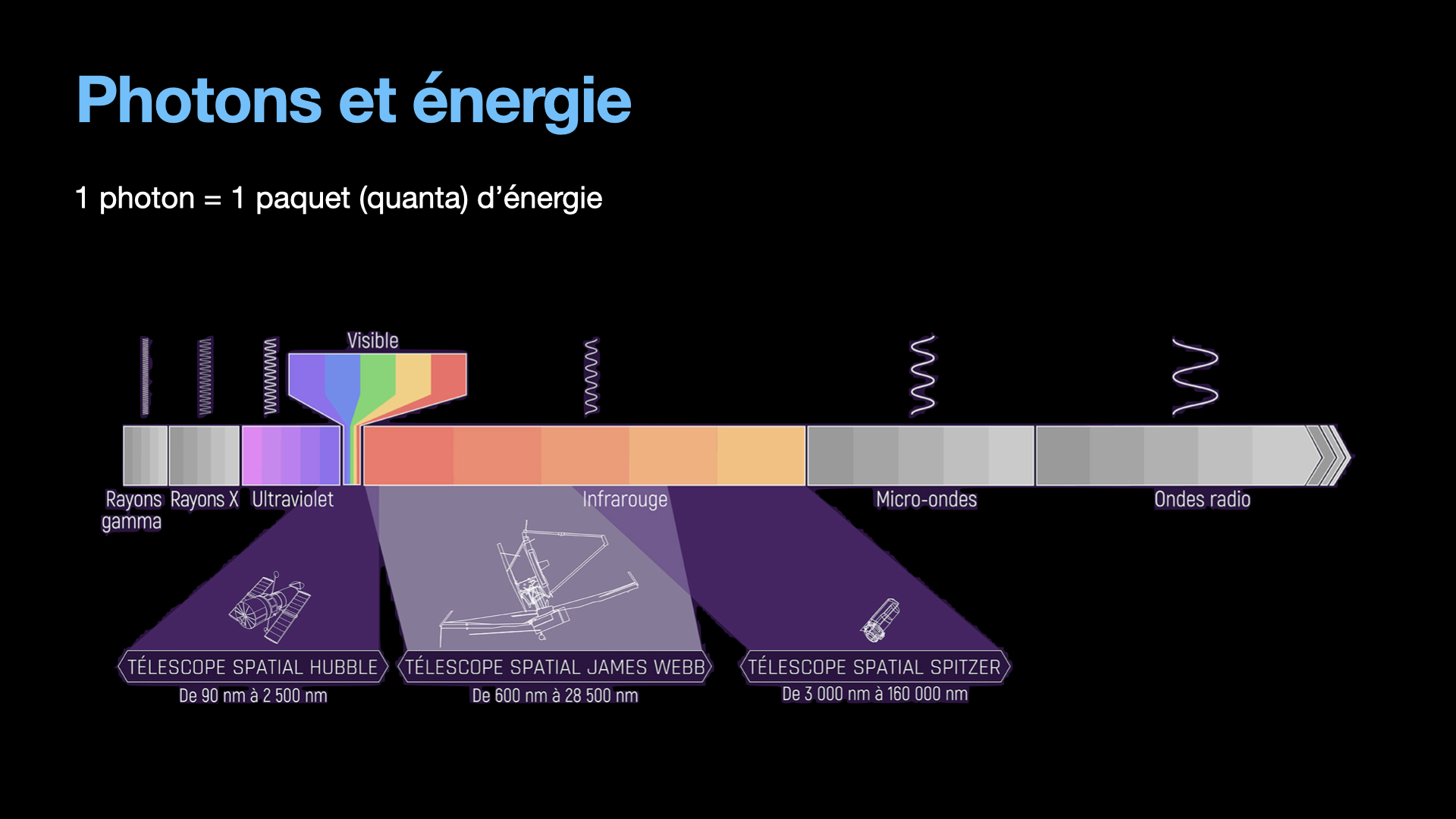
Anyway, back to Einstein. Einstein also worked on interactions between the photons and matter, which will eventually become the cornerstone of Lasers, 50 years later. Einstein will describe 3 kind of interaction between photons and matter. 4 if you count what happens when a photon doesn’t interact with matter, which is kind of important as well.
To understand how it works, let’s start with the description of an atom. As you may know, atoms are composed of a nucleus, itself composed of neutrons and protons. Around this nucleus are electrons. Bohr, a scientist from the early 20th century, developed a view of the electrons and especially the way electrons go around the nucleus by describing orbits. No too different to the solar system, with the nucleus at the center, and the electrons turning around the nucleus along circular orbits. Each atom has a given set of orbits, that depends on the type of atom (orbits from a Carbon atom are different from an Hydrogen atom for instance, but two carbon atoms have the same orbits…) With time, we will discover that this view is a little naive, and electrons are actually kept in what are called orbitals, which are more like clouds around the nucleus, in 3D, with various shapes. But Bohr’s view is good enough for our discussion here. So, electrons are moving around the nucleus. Good.
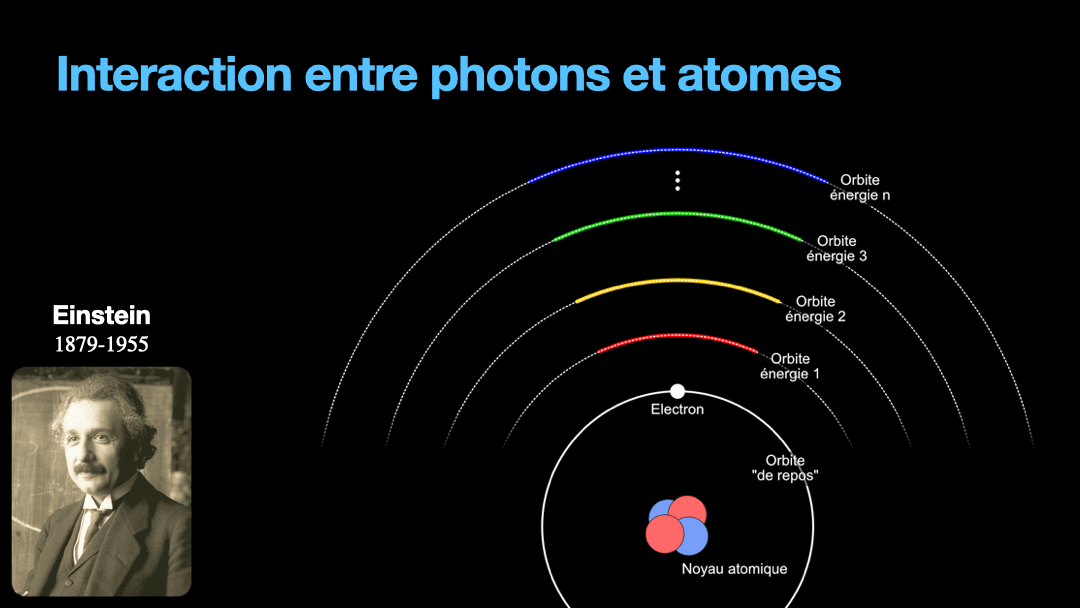
It is important to know that atoms can store energy. if you imagine an atom with the electrons as close as possible to the nucleus, this atom is at a state that we call the ground state. The lowest energy state. This atom can capture and store energy. The energy is stored by moving an electron from an orbit to another. The electrons are moving away from the nucleus, storing more energy. Eventually, we can give so much energy to an atom that the electron goes so far away from the nucleus that it is no longer tied to that nucleus. The atom loses an electron. It has become ionized…
Remember how I described a photon as a packet of energy? Well, turns out that atoms can capture energy by absorbing photons. Given an atom at ground state, if a photon that carries the exact amount of energy to allow an electron from that atom to move from one orbit to another has a chance of being captured and stored by that atom…. Amazing, right? This process is called absorption. If the photon doesn’t carry the right amount of energy for a transition, then the photon passes through the atom without interaction.
Then, we have an atom with an electron that is in a high orbit. This atom is energized. As with many things in physics, this is not a stable state and the atom desperately needs to let that energy go at some point. So eventually, the electron is going to go the opposite direction, and move toward the nucleus, back to it’s original orbit. In doing so, it must shed the excess energy. Energy is never destroyed nor created, it is transformed (as the famous say goes from Lavoisier). And of course, in the process of loosing this energy, the electron generates a photon with the exact same energy than the transition that the electron is doing. This process is called spontaneous emission. It is probabilistic, that is to say that the emission is going to happen at a moment that we can’t chose. We can only know that after 3ms, 50% of the electrons have made the transition for instance. I write 3 ms but it can be 10ms or it can be 3ps, or whatever. It depends on the type of atom and also other factors.
Let’s go back to Einstein. He explained that light and matter can have 3 kind of interaction, as I wrote earlier. The first interaction is the absorption of light. A photon with a given energy is approaching an atom at rest. This atom has a possible electronic transition that is exactly the same energy than the photon is carrying. In this case, the atom will “capture” the photon. The energy of the photon will be used to move an electron from an orbital to the other, corresponding to the exact energy from the photon. Remember that energy is never lost, so if a photon transports a slightly different amount of energy approaches the atom, nothing will happen because the atom can’t just use a bit of the energy of the photon and leave the rest “be”. This interaction is called absorption, pretty obvious.
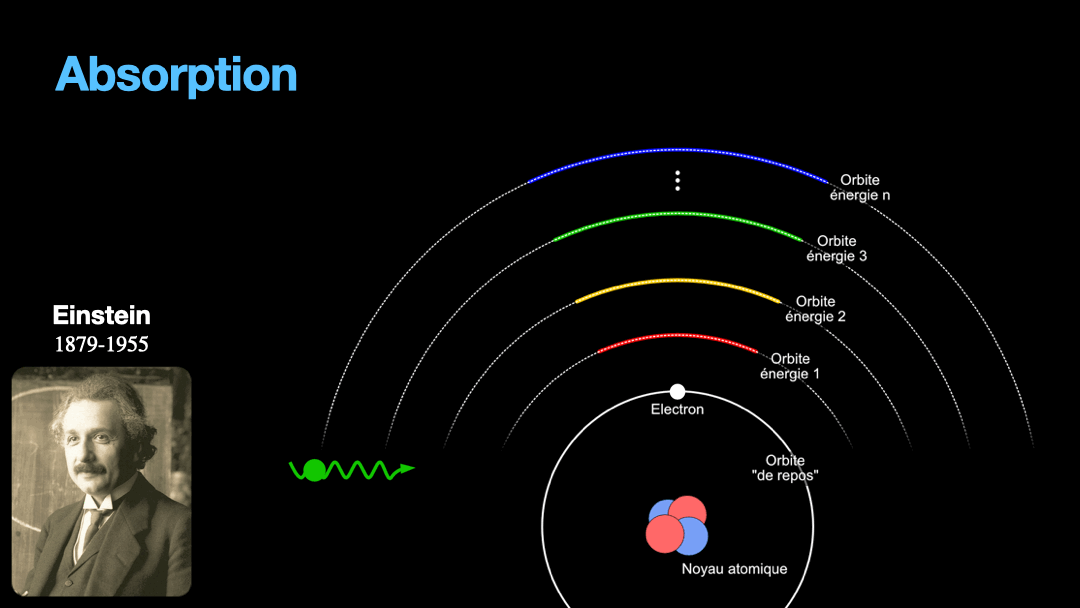
The second interaction between light and matter is the opposite of absorption. Like I wrote earlier, an atome that is energized can’t stay forever that way. It will eventually shed the excess energy and fall back to it’s rest state. In doing so, the atom will emit a photon that is the exact same energy than the electronic transition done by the electron going back to rest. Again, this is something that is probabilistic, one can’t predict when a photon will be emit, we can only say that within a given amount of time, 50% of a bunch of atoms that were excited will have emitted a photon. This phenomenon is called spontaneous emission.

One important point to make here is that the direction of the emission of the photon is totally random. The photon is effectively a different photon from the one that got absorbed and will be generated going in a totally random direction….
The final and honestly most important interaction is at the core of the laser. It is called stimulated emission. Like spontaneous emission, everything starts with an energized atom. This atom has an electron in an orbital ready to be emitted spontaneously and ready to give a photon at some given energy corresponding to the electronic transition. What is different from the spontaneous emission is the presence of a photon with this exact energy at the start of the interaction. This photon is traveling toward the excited atom. And as soon as the photon is close enough to the atom, the mere presence of the photon will trigger the electron to go down, and emit another photon which will be the exact copy of the initial photon. We therefore end up with two twin photons when there was only one at the start of the interaction. The reason it happens is because photons are also called bosons. Bosons, on the contrary to particles like electrons and protons, love to aggregate to one another. So the more bosons at one place, the more it attracts other bosons to that place basically. When trying to move two protons together is tough because they repel. But I go a little too far, this is not important here.

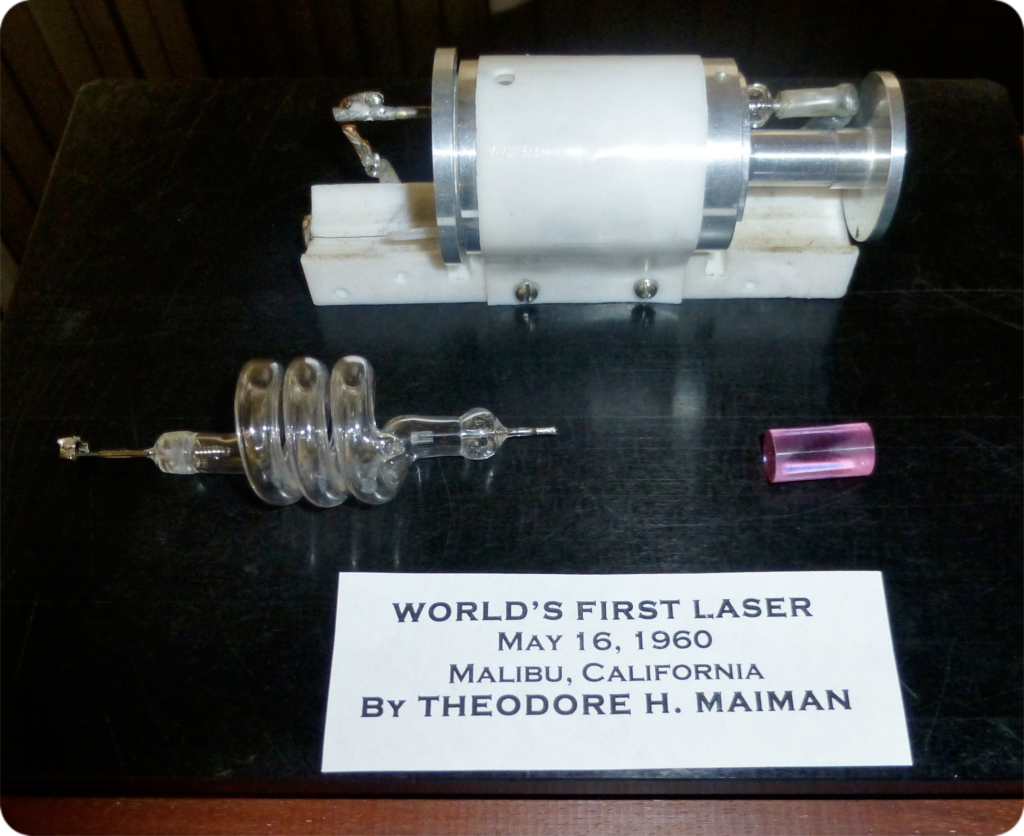
In 1951, Townes creates the first Maser, which is a microwave laser technically. It was easier at the time. For this feast, he will obtain the Nobel prize along with other scientists. The first real laser with light will be created in 1960 by Mainman and this is the start of the era of lasers. His laser used a ruby as the amplification medium, that is to say the atoms that will be excited and emit photons.
And now, all of this is nice and interesting, at least I hope. But what is the point? what is the advantage of lasers compared to a candle or a tungsten lamp?
Until the laser, light was created mostly by thermal sources. Fire obviously, and candles are emitting light from the heat it produces. Lamps actually were also producing light from thermal effect. A tungsten filament is heated high enough to become white. These types of light are emitting a large amount of photons that are all different from one another, as can be seen in the emission spectrum. Moreover, these photons are going in all directions with no order whatsoever. To focus this kind of light is difficult because of the large emission spectrum (lenses typically have chromatic aberrations, and a light source with a large emission spectrum will be strongly affected by those). Not to mention the weak energy from these light sources.
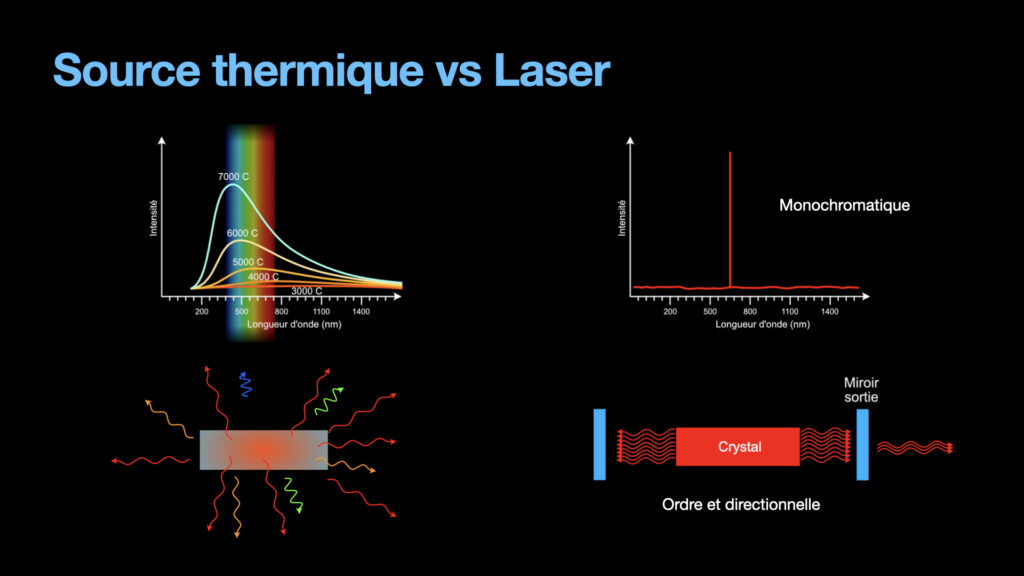
A laser on the other hand is monochromatic, the photons from the laser beam have only one wavelength (in theory, in reality, they have a very very small bandwidth, but nothing compared to a thermal light). The photons are all going in the same direction, and they are ordered, all in phase. All of this help to focus al laser light to tiny diameters and reach energy densities that allow to melt and even vaporize material. This is the reason why lasers are so important nowadays.
[1] – https://en.wikipedia.org/wiki/Emission_theory_%28vision%29